Catalogar
In minimally invasive surgery, the insufflator’s pressure control and monitoring are pivotal to patient safety and optimal operative visualization. Selecting the appropriate pressure sensor involves balancing high-precision measurement, rapid dynamic response, and enduring stability. This article provides a comprehensive analysis of MEMS barometric sensor materials, packaging methods, and manufacturing processes, covering functional requirements, critical parameters, installation and soldering best practices, pre-production testing workflows, and safety and reliability validation strategies. Aimed at engineers and technical purchasers, it offers a systematic approach to determine the ideal WF100E analog pressure sensor solution under rigorous clinical and regulatory environments, ensuring device performance integrity even after extensive use and sterilization.
Detailed Performance Requirements
1.1 Accuracy and Linearity Standards
Insufflators require sensors with accuracy better than ±0.5%FS to tightly control the discrepancy between displayed and actual intra-abdominal pressures. High linearity ensures proportional response across the measurement range, reducing calibration complexity and enhancing system reliability.
1.2 Sensitivity and Dynamic Responsiveness
Rapid pressure changes caused by tissue manipulation or tubing occlusions demand millisecond-level response and high pressure resolution. MEMS microstructures, through minimized diaphragm dimensions and optimized microchannels, achieve swift detection of subtle pressure variations, safeguarding the surgical procedure.
1.3 Long-Term Stability and Durability
Repeated pressure cycles and sterilization challenge sensor longevity. Premium MEMS sensors utilizing silicon or low-expansion ceramics combined with full-weld or laser-weld sealing maintain zero drift below ±0.1%FS over time, ensuring performance consistency throughout the medical device lifecycle.
1.4 Materials and Packaging Techniques Comparison
- Diaphragm Choices: Silicon offers ultra-high sensitivity whereas stainless steel excels in mechanical robustness.
- Sealing Methods: Ceramic substrates with laser welding deliver superior corrosion resistance; all-welded metal housings provide maximum structural protection and EMI shielding.
- Leak Prevention: Composite O-ring and metal gasket assemblies block ingress of fluids and sterilization agents.
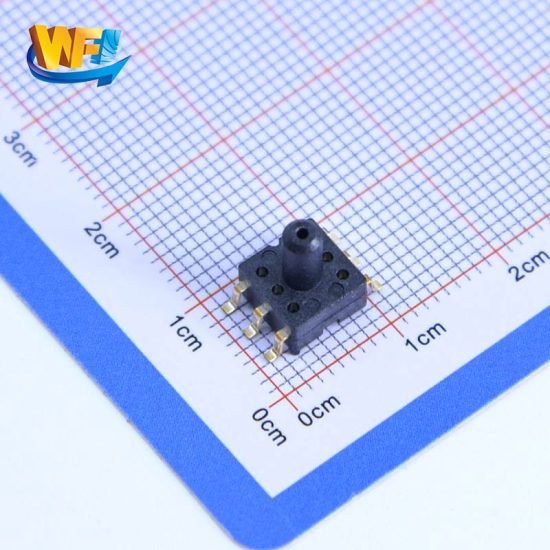
Critical Selection Parameters Explained
2.1 Range Matching and Safety Margin
With clinical pressures up to 20 kPa, select a sensor range of approximately 25 kPa to include at least 20% safety margin, preventing element overload and preserving full-scale linearity.
2.2 Resolution and Minimum Detectable Increment
Resolution of 0.1 kPa or better is necessary for fine pressure adjustments, feeding detailed data to control algorithms for precise insufflation.
2.3 Temperature Compensation and Environmental Tolerance
Built-in compensation circuits limit thermal drift to ±0.01%FS/℃ between 20℃–40℃, maintaining stability against ambient variations commonly found in operating rooms.
2.4 EMC and Interference Resistance Standards
Medical settings are rife with electromagnetic noise sources; sensors must incorporate EMI shielding, input filtering, and differential outputs, along with PCB grounding and layout practices to ensure low-noise, repeatable measurements.
Installation and Soldering Best Practices
3.1 Optimal Placement and Tubing Configuration
Mount the sensor near the gas inlet to minimize tubing-induced distortions. Ensure gradual bends and avoid sharp angles to prevent flow turbulence and erroneous readings.
3.2 Controlled Soldering Temperatures and Moisture Exposure
Use low-temperature solder alloys (melting point ≤ 250℃) and step reflow techniques to prevent internal adhesive degradation. Control humidity during soldering to avoid moisture absorption by sensitive components.
3.3 Lead Stress Protection Strategies
Conduct post-solder tensile tests on leads, then reinforce joints with silicone or epoxy compounds to distribute mechanical stresses and prevent fatigue failures during transport and use.
3.4 Electrical Sealing and Shielding Procedures
Apply conformal coatings to electrical connections, integrate grounded shields, and perform final potting to fully isolate internal circuits from external environments, raising the protection rating to IP67 or higher.
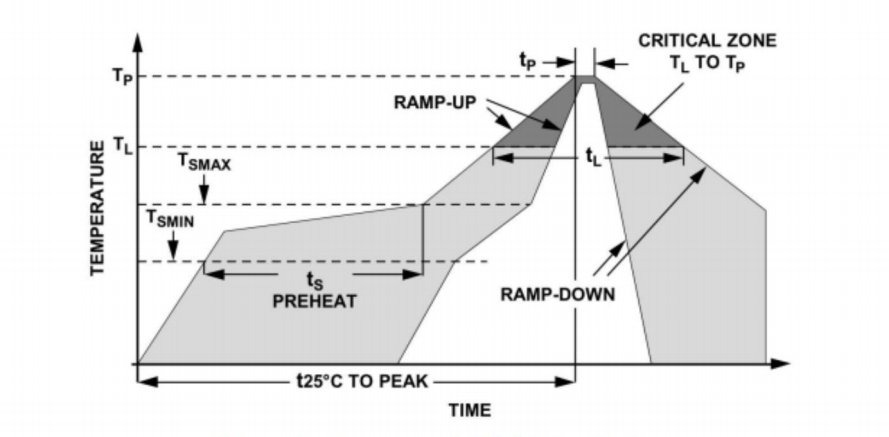
Pre-Production Testing Protocols and Tooling
4.1 Multi-Point Calibration and Reference Equipment
Deploy automated pressure calibration rigs or deadweight testers for zero, half-scale, and full-scale calibration. Generate statistical calibration datasets to verify batch consistency and accuracy distribution.
4.2 Output Characteristic Profiling and Error Metrics
Utilize precision DAQ systems and custom jigs to perform pressure sweeps, capturing hysteresis and repeatability. Calculate metrics such as nonlinearity, histéresis, and repeat accuracy to confirm performance within ±0.5%FS limits.
4.3 Environmental Aging and Accelerated Life Testing
Subject units to 85℃/85%RH cycles and vibration profiles to simulate long-term usage. Measure drift in zero offset and sensitivity post-aging to model real-world lifespan performance.
4.4 Essential Laboratory Instruments and Automation Integration
Equip facilities with high-resolution digital multimeters, Wheatstone bridge circuits, climatic chambers, and PLC/PC-controlled test platforms. Implement software for real-time data acquisition, analysis, and automated pass/fail sorting.
Comprehensive Safety and Reliability Validation
5.1 Redundant Sensing and Fail-Safe Design
Integrate dual or multiple sensors with voting logic for data redundancy; upon primary sensor fault, switch to backup channels and issue alerts, maintaining uninterrupted pressure monitoring.
5.2 Long-Term Cycling and Fatigue Analysis
Execute thousands of pressure cycles under simulated clinical conditions, monitoring parameter drift and fatigue-induced deviations to inform maintenance schedules and service intervals.
5.3 Overload Tolerance and Shock Testing
Perform static overload tests at 1.5–2×FS and multi-axis shock per IEC 60068-2-27 to verify diaphragm resilience and package integrity under extreme loads.
5.4 Regulatory Compliance and Medical Certification
Adhere to ISO 13485 quality management requirements and compile design verification, risk assessment, and biocompatibility documents for CE marking and FDA clearance, ensuring full regulatory alignment.
Conclusión
This guide offers a thorough methodology for selecting the WF100E analog MEMS pressure sensor for medical insufflators, covering advanced material choices, precision performance metrics, refined installation and soldering processes, rigorous pre-production testing, and stringent safety validation. Employing these best practices ensures that insufflators deliver accurate, reliable, and safe pressure control, ultimately supporting successful minimally invasive surgeries and patient well-being.
La introducción anterior solo rasca la superficie de las aplicaciones de la tecnología del sensor de presión. Continuaremos explorando los diferentes tipos de elementos de sensores utilizados en varios productos., Cómo funcionan, y sus ventajas y desventajas. Si desea más detalles sobre lo que se discute aquí, Puede consultar el contenido relacionado más adelante en esta guía. Si está presionado por el tiempo, También puede hacer clic aquí para descargar los detalles de estas guías Producto del sensor de presión de aire datos PDF.
Para obtener más información sobre otras tecnologías de sensores, por favor Visite nuestra página de sensores.